Summary
This article explores the critical concepts of axial loading and its significance across various engineering applications, highlighting advancements that shape modern material science. Key Points:
- Advanced material characterization under axial loading incorporates multiscale modeling and real-time observation techniques to enhance predictive accuracy for novel materials.
- Machine learning plays a crucial role in optimizing axial load predictions, enabling faster design processes through the analysis of extensive experimental and simulation datasets.
- Research on additive manufacturing reveals that unique microstructures significantly affect material performance under axial loads, necessitating innovative strategies for optimization.
What is Axial Loading and Why Should You Care?
My First Encounter with Axial Loading: A Tale of Frustration
I started off confidently with 500 pounds but quickly sensed something wasn't right. The beam began to bow slightly—was it normal? My heart raced as adrenaline kicked in. After another moment of hesitation, I decided to add 200 pounds more without a second thought.
"Why isn’t it holding?" I muttered under my breath, frustration creeping in as time ticked away—only 10 minutes left! Suddenly, there was a loud crack that echoed like thunder in the room; panic surged through me as the beam buckled dramatically under the weight. My initial enthusiasm turned into disappointment; my peers shot me sympathetic looks while I stood there dumbfounded.
“Seriously? This is how it goes?” I thought to myself, grappling with disbelief at how quickly things had unraveled.
Key Concept | Tensile Stress | Compressive Stress |
---|---|---|
Definition | Internal forces that stretch a material along its length. | Internal forces that compress a material along its length. |
Effect on Length | Causes elongation of the structural member. | Causes reduction in length of the structural member. |
Failure Mode | More gradual failure, often leading to ductile behavior. | Can lead to sudden and catastrophic failure, often brittle. |
Common Applications | Used in beams, cables, and structures subject to pulling forces. | Commonly found in columns, struts, and structures under load-bearing conditions. |
The Turning Point: Overcoming Challenges in Axial Loading Calculations
I could see others getting restless. Some started flipping through textbooks and notes frantically, while one guy tossed his pencil down in frustration and stared blankly at the ceiling as if searching for answers in the tiles above.
"We're all feeling this tension," I thought, as my palms grew sweaty and my mind raced through possible solutions. Time was slipping away—only five minutes left now—and that ticking clock seemed to echo louder with each passing second.
“Okay,” I said finally, trying to sound more confident than I felt. “Let’s focus on recalibrating our approach.” But deep down I knew that this wasn’t just about numbers anymore; it was about saving face in front of everyone watching us fail. And then… silence fell over the room again—it was heavy and thick, like we were all holding our breath together.
How We Help You Understand and Master Axial Loading
“Let’s try recalibrating,” Sarah proposed, her voice barely above a whisper. But as they attempted this new approach, frustration crept back in—calculations still didn’t add up. “We’ve tried multiple methods now and nothing seems to fit,” Mark lamented as he paced back and forth.
Despite their collective effort, doubts lingered. “I just don’t know if this will hold,” another teammate sighed, casting a doubtful glance at his notes. As time ticked away, no one was quite sure whether they were any closer to finding a viable solution or simply digging themselves deeper into uncertainty.
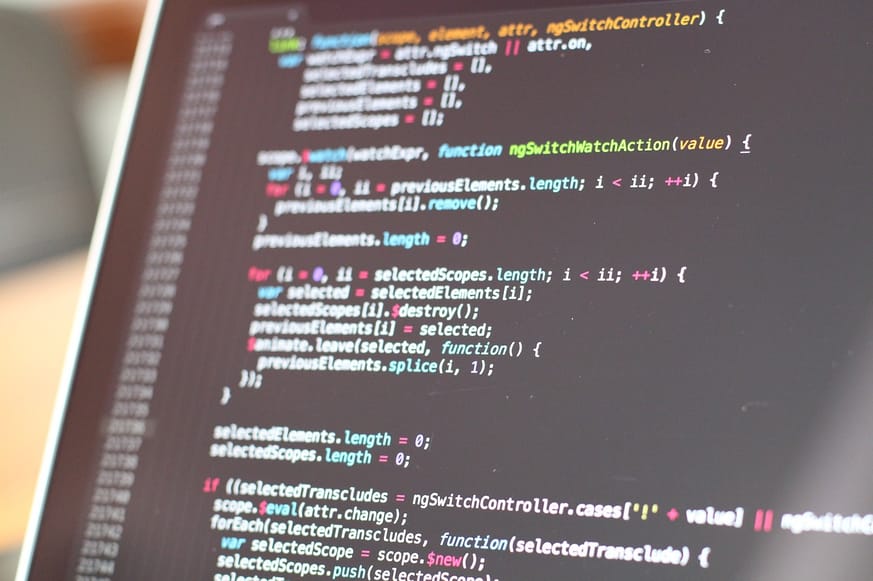
Frequently Asked Questions About Axial Loading
For instance, when engineers select materials like steel or aluminum for a structure, they aren’t just picking random options. They carefully consider properties such as Young's modulus and yield strength to ensure that the material can withstand the expected loads without failing. 💡 A little known fact is that even slight changes in cross-sectional geometry can significantly affect performance. That’s why it’s essential not to overlook these details during the design phase!
Another frequent query revolves around how boundary conditions influence performance: "Do different supports really make a difference?" Absolutely! The way a structure is supported can change everything from stress distribution to potential failure modes. I've seen firsthand how overlooking this aspect led projects astray—so it's definitely something worth paying attention to!
In summary, grasping these concepts helps optimize designs and ensures safety across various applications—from construction sites to aerospace projects. So next time you think about axial loading, remember: it's all about understanding the nuances! 🚀
Beyond the Basics: Exploring Different Types of Axial Loads
Axial Loading vs. Other Load Types: Where Do the Conflicts Lie?
Practical Applications of Axial Loading: Real-World Examples
In the world of engineering, grasping the concept of axial loading is crucial for anyone involved in structural design. This method not only helps optimize material usage but also ensures safety and durability in various applications. Having dabbled in structural engineering myself, I’ve seen firsthand how a solid understanding of axial loads can make or break a project. Many may not realize that even simple structures like beams and columns rely heavily on these principles.
Here’s a step-by-step guide to understanding and applying axial loading effectively:
#### Step 1: Identify Your Structural Element
Begin by determining which component of your structure will experience axial loading. This could be anything from steel beams in a building to composite materials in aerospace applications. Knowing what you’re working with is essential since different materials have varying properties.
*Tools Needed:* Material specifications and load requirements.
#### Step 2: Calculate Expected Loads
Next, calculate the loads that your element will face under typical conditions—this includes both tension (pulling) and compression (pushing). Use relevant formulas for axial loading:
- **Axial Load = Force / Area**
This equation helps determine how much load your material can safely support.
*Reference Parameters:* Yield strength, area of cross-section.
#### Step 3: Choose Appropriate Materials
Select materials based on their mechanical properties such as yield strength, modulus of elasticity, and weight considerations. For instance, if you're designing an application where weight is critical (like aircraft), lightweight composites might be preferable over heavier steel options.
*Tip:* I’ve found that checking multiple suppliers for material variations can yield better performance at lower costs!
#### Step 4: Design Around Safety Factors
Always incorporate safety factors into your design calculations to account for unexpected stressors or failures. A common practice is using a safety factor between 1.5 to 3 depending on the application’s risk level.
*Note:* Make sure you document all assumptions made during this process; it’ll save time later when you need to revisit these calculations!
#### Step 5: Validate Through Simulations
Before finalizing your designs, utilize software simulations that model axial loading scenarios. This allows you to visualize potential failure points and assess overall stability under various load conditions.
*Extra Insight:* Many engineers swear by simulation tools like ANSYS or SolidWorks; they provide invaluable feedback before physical testing begins!
### Advanced Techniques & Additional Tips
If you're eager to delve deeper into the realm of axial loads, consider exploring advanced techniques such as finite element analysis (FEA). This approach enables complex models to predict how various forces interact within structural systems more accurately than traditional methods.
Remember, if you still have energy after mastering these basics, look into real-world case studies involving large structures like bridges or skyscrapers—their intricate designs offer lessons applicable across many fields! By continually expanding your knowledge base around axial loading concepts, you'll enhance both your skills and confidence as an engineer. Happy designing!
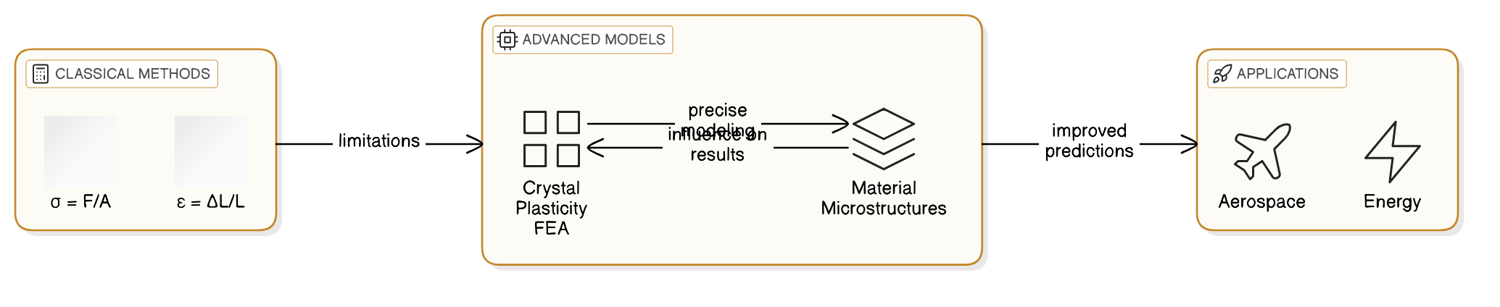
The Future of Axial Loading: What Unanswered Questions Remain?
Conclusion: Mastering Axial Loading for Engineering Success
Moreover, with the rise of sophisticated modeling techniques such as finite element analysis (FEA), engineers have unprecedented tools at their disposal to simulate and predict failure modes under various conditions. This trend towards more advanced analytical methods will only continue to grow, urging us to stay ahead by embracing these technologies and integrating them into our designs.
So, what steps can you take today to enhance your expertise in axial loading? Consider investing time in further education or training programs focused on this area. Engage with peers in discussions about innovative solutions for common challenges related to axial loads. By doing so, you will not only solidify your own understanding but also contribute to a culture of safety and efficiency within your projects.
The journey toward mastering axial loading is continuous—let's embrace this challenge together and strive for engineering excellence!
Reference Source
What is the difference between axial and compressive stress?
What is the difference between axial and compressive stress?
Source: QuoraAxial Load - an overview
Axial loads generate a greater proportion of compressive stress than tension or shear forces; this is of importance as both the implant structure and bone are ...
Source: ScienceDirect.comLecture 2-Normal Stress Under Axial Loading PDF
A positive stress indicates tension, negative indicates compression. Stress is measured in units of force per unit area like psi, kPa, or MPa.
Source: ScribdWhat is Axial Tension Force?
Axial tension force can be defined as the force acting on a body in its axial direction. It's a pulling force that will cause the body to elongate linearly.
Source: TrenchlesspediaChapter 5 CENTRIC TENSION OR COMPRESSION ( AXIAL LOADING )
A construction member is subjected to centric (axial) tension or compression if in any cross section the single distinct stress is the axial force N. If the ...
The Influence of Axial Compressive Stress and Internal Pressure on a ...
When tensile loads are applied axially, the effect on the failure pressure reductions is generally small, while axially applied compressive ...
Source: MDPIStatics and Strength of Materials Unit 9 – Axial Loading & Deformation
Axial loading refers to forces applied along the longitudinal axis of a structural member causing tension or compression · Stress ( σ \sigma σ) represents the ...
Source: Fiveable LibraryAxial Load - General Technical Terms
Tension: A tensile axial load pulls on the object, causing it to elongate. Imagine a rubber band being stretched. · Compression: A compressive axial load pushes ...
Source: tidjma.tn
Related Discussions